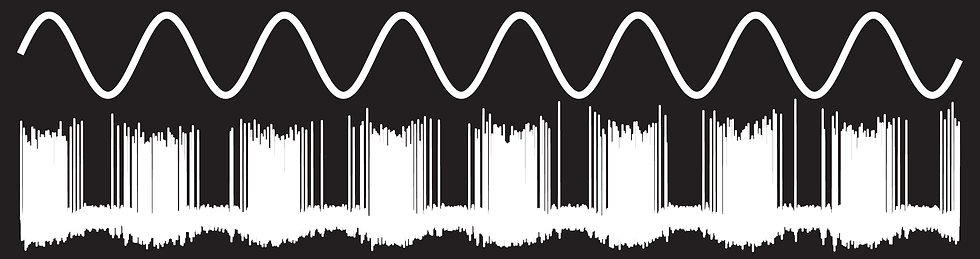
General Interests
I am interested in how the brain controls behavior. Specifically, I want to discover the neurophysiological mechanisms by which brains detect and interpret sensory information. My approach uses quantitative analyses of the animal’s natural behaviors as a tool to reveal the meanings of neural codes.
I study weakly electric fish because these animals have unique adaptations that facilitate the study of brain mechanisms. Using their electric organ, these fish generate complex social signals that are easily recorded, reproduced, and modified. The social signals can be described and expressed via computational models, which in turn can be used to decode sequences of action potentials from electrophysiological recordings of brain activity.
My research has revealed critical insights into how non-linear computations are used to extract information and features of sensory signals, including a category of signal known as ‘envelopes’ that are also important features of acoustic and visual cues. More recently, I’ve shown how the nervous system alters these computations in response to changes in the animal’s sensory world.
Current Research
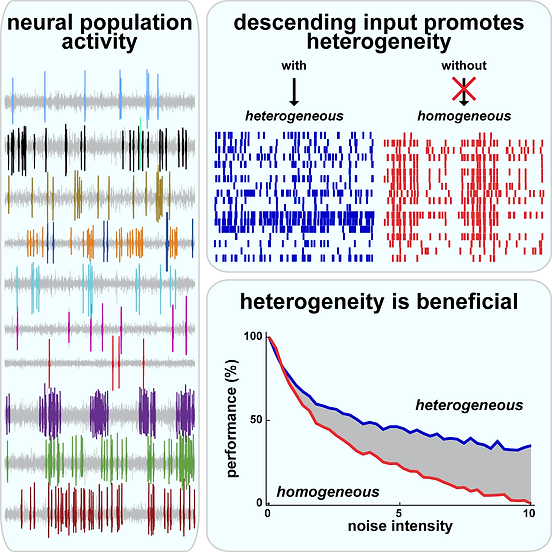
The role of feedback in response heterogeneity
Highlights
-
Response heterogeneity within sensory neural populations serves a beneficial function
-
Pharmacological inactivation of feedback strongly reduces heterogeneity
-
Decoding is more robust to noise addition before feedback inactivation
-
Heterogeneity is actively promoted in sensory systems to benefit coding
Neural systems are adapted to their environment in order to optimally encode natural sensory stimuli with a given set of statistics. The mechanisms underlying this adaptation are poorly understood in general. Studies in an array of sensory systems have shown that neural responses are optimally tuned utilizing various strategies to efficiently process sensory information enabling matched behavioural responses to certain stimulus statistics.
The goal of this study is to ask what happens to neuronal and behavioral responses when stimulus statistics change over time? A combination of electrophysiology, behavioural paradigms, and pharmacology is used to uncover at what level (single neurons vs population) adaptation takes place, as well as the underlying mechanisms and neural circuits that are involved.

Sensory Adaptation
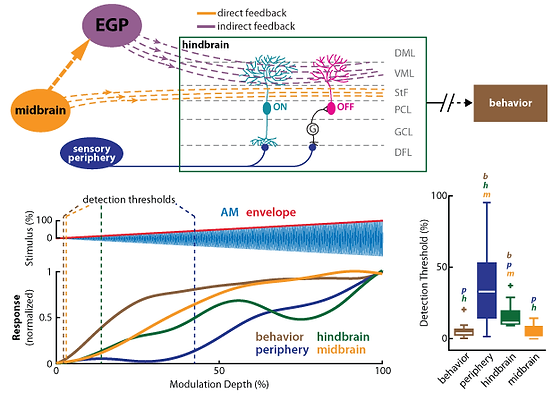
Growing evidence suggests that sensory systems are adapted to optimally encode incoming sensory stimulus information found in natural environments. However, natural stimuli often have complex stimulus statistics, in which individual stimulus features must be separately extracted and transmitted in ascending pathways. There is much evidence that sensory neurons one synapse away from the periphery can already deconstruct complex stimulus features and are capable of optimally transmitting this information through processes such as temporal whitening.
The goal of this project is to understand the role of descending pathways during sensory threshold determination. Furthermore, if these extensive feedback pathways do play a role in optimizing neural responses, what are the underlying mechanisms of each descending pathway? Finally, how do these optimized responses translate to the behavioral perception of the animal?
The Role of Feedback in Determining Sensory Thresholds
Envelope Coding in Sensory Systems
The goal of this project is to understand how envelopes (i.e., second order stimulus features) are represented across successive stages of processing in the electrosensory system in order to lead to behavior. This includes looking at the periphery to see what strategy is used (single or population coding), and then move on to higher brain areas such as the hindbrain or the midbrain.


Phase Invariant Representation of Electro-communication Signals
The goal is to identify the neuronal mechanisms that allow a phase invariant representation of electro-communication signals (i.e. chirps) across different brain areas within the electrosensory system.
Past Research
Bionics
The goal of this project within the framework of the DFG funded Graduate School Bionic (GK 1572) was to design a bionic electrolocation sensor for coronary diagnostics. Together with Dipl. Biol. Martin Gottwald, M.Sc. Kavita Mayekar (FEM modelling) and Dr. Herbert Bousack (Forschungszentrum Jülich) we started to develop a catheter-based sensor system working according to the principles of active electrolocation. The sensor was intended to be used for routine blood vessel diagnostics that could also be adopted for finding and analyzing pathologic changes of (coronary) artery walls.
Curtesy of Kavita Mayekar.
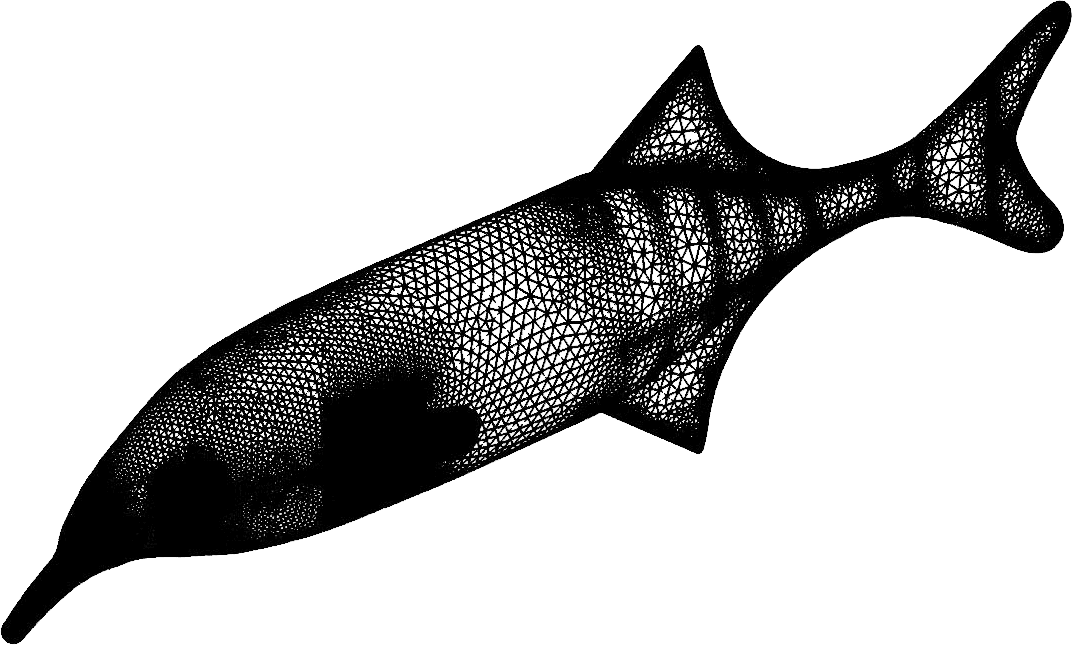
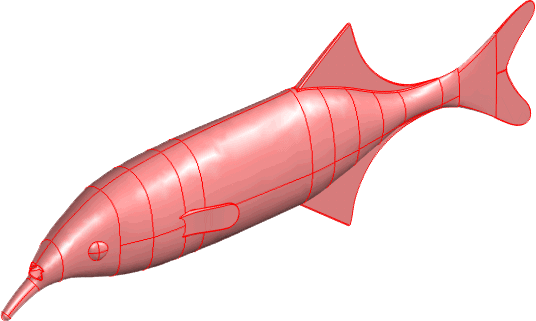

Anatomy
In a project carried out at the University of Missouri St. Louis, MO, USA, I investigated the descending projections from the midbrain to motor control centres in the brain stem of the paddlefish (Polyodon spathula). Under supervision of Dr. Michael Hofmann I gained experience in the use of anatomical methods and the analysis of such data. As methods, I did tracer injections (BDA) into the brain stem and analyzed the neurons labelled in the midbrain according to their morphology and their relative positions. The aim of this study was the identification of midbrain nuclei that are involved in the generation of specific motor behaviours.
Physiology
During my doctorate, I was interested in the coding strategies of the first central stage of the ascending electrosensitive pathway, the electrosensory lateral line lobe (ELL) using the weakly electric mormyriform fish Gnathonemus petersii. My goal was to study the spatial dimensions of receptive fields (RFs) of electrosensitive neurons of the ELL with respect to two proposed electrical foveae (i.e. the Schnauzenorgan and the head) in this fish. In contrast to most sensory systems, my results show that RF sizes did not depend on their position on the receptive surface. Instead of size, the complexity of the RF structure seems to be more important and constitute a filter of sensory input. These findings support the present hypothesis of pre-receptor mechanisms, which are crucial for the electrosensory system.
A further goal of my thesis was to find out in which way neurons encode important object parameters. My results suggest that contrary to the primary afferents, ELL neurons do not only use a temporal, but also a rate code to encode the material of an object. This possibility would allow for a high spatial resolution to encode several stimulus parameters together with a fast processing.
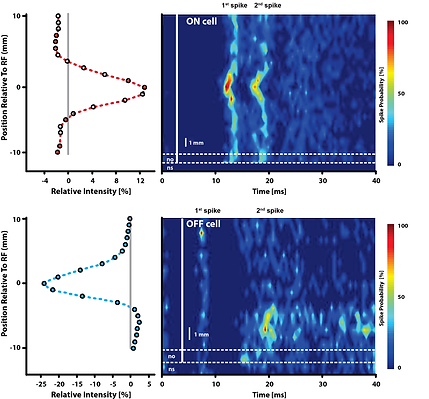